Tool 6.7 Soil health benchmarks and guidelines for managing problem soils
Soil pH (acid soils)
Soil pH is a measurement of potential hydrogen ions within the soil. Soil pH tests are completed by extracting hydrogen using water (H20) or calcium chloride (CaCl2), but results from the CaCl2 test are more consistent so are the recommended measures to be used in lab soil test results.
A pH(H20) of 7.0 is considered neutral (above 7 is alkaline) so, strictly speaking, any soil with a pH below 7 is acidic. However, most plants have maximum efficiency of nutrient uptake in slightly acid soils, so a neutral pH isn’t necessarily the target for most agricultural production.
Most plants and micro-organisms have defined ranges of pH for optimal growth. The optimal range for plants is generally between 5.5 and 8.0 (pHCaCl2) whereas most soil organisms function best between pH 6.0 and 7.0. All species will grow outside their pH limits, but productivity and persistence may be less than their potential.
Table 6.3 below shows the preferred soil pH ranges for common pasture species.
Table 6.3 Preferred pH range for some common pasture species
Plant species |
Soil pH (CaCl2) |
Lucerne |
5.3–7.3 |
White clover |
5.3–6.3 |
Sub clover |
4.5–6.3 |
Phalaris |
5.0–7.8 |
Perennial ryegrass |
4.6–6.3 |
Annual ryegrass |
4.6–6.3 |
Cocksfoot |
4.3–6.8 |
Many plants prefer slightly acidic conditions. Plant roots are often affected by aluminium (Al) and manganese (Mn) toxicities as these elements are more soluble in strongly acid soils or when pH(CaCl2) falls below 5.0. Table 6.4 below shows the aluminium concentrations at which growth is reduced by 10% for common introduced pasture species.
Table 6.4 Critical aluminium concentrations for growth
Soil test level above which yields are reduced |
|||
Species |
Aluminium (% of Cation Exchange Capacity) |
0.01M CaCl2 (mg/kg) |
Sensitivity |
Lucerne |
5 |
2 |
highly sensitive |
Red clover |
10 |
4 |
sensitive |
Woolly pod vetch |
20 |
8 |
moderately tolerant |
Oats |
30 |
13 |
highly tolerant |
Native species, such as wallaby grass/white top (Austrodanthonia spp), weeping grass (Microlaena stipoides), kangaroo grass (Themeda triandra), redgrass (Bothriochloa macra), and windmill grasses (Chloris spp.) are not included as they are rarely sown and limits are not as well defined. Weeping grass is able to tolerate soils with low pH. The many sub-species of wallaby grass have different preferred pH ranges. Kangaroo grass and redgrass grow on low to neutral pH soils, while windmill grasses prefer heavier neutral to alkaline soils.
Managing acid soils
A sustainable target soil pH(CaCl2) in the topsoil (0-10 cm) is minimum 5.5 as this not only removes any plant yield constraints but prevents acidity issues from increasing in the subsurface soil (10-20 cm). The carbonate within lime reacts with hydrogen ions within the soil to create bicarbonate ions which neutralises and removes excess hydrogen ions. Bicarbonate movement is controlled by soil pH and occurs at pH levels greater than 5.5. Physical movement of fine lime particles (less than 0.8 micron) can also occur by washing down macropores. Lime movement is considered slow, approximately 1-3 cm per year and therefore incorporation by cultivation is encouraged. The amount of lime required to address acidity is dependent upon current soil pH (figure 6.19), target pH, soil pH buffering capacity (that is the amount of lime required to change pH by 1 unit) and lime quality. Soil pH buffering is influenced by cation exchange capacity (CEC) which in turn is influenced by soil texture and organic carbon levels. Sands will respond more quickly to lime than soils with higher CECs, such as clay soils.
Figure 6.19 Amount of lime required (t/ha) to lift pH(CaCl2) in top 10 cm of soil.
Liming is a necessary input to counteract the acidification occurring within soils but it can be expensive, especially if the farm is a long way from the quarry. In weighing up the investment of lime on paddocks consider the following questions:
- Is my topsoil pH above 5 at 0-10 cm? If yes, pasture growth response is less likely.
- Is subsurface acidity above 5 (10-20 cm)? If yes, pasture growth response is less likely.
- Is my subsoil (>20 cm) acid? This is not usually practical or economic to overcome. In some cases, subsoils are naturally acidic but for those that are not, prevention becomes an important goal.
- Is the pasture tolerant of the current levels of aluminium within the soil? If yes, a lime response is unlikely.
- Is there something else (e.g., phosphorus) that is the most limiting factor? If yes, a lime response is unlikely to be maximised. In turn the pasture response to fertiliser may also be reduced due to poor root growth.
Strategies producers use in liming to maintain good soil pH are:
- Apply lime to a portion of the farm each year, rather than lime the majority of the farm every 7 to 10 years.
- If high rates are needed, split over a few years (e.g., 3 to 5) rather than in one large application.
- Apply to reduce a tax bill or in good years.
- Prioritise paddocks to be sown or are sown to desirable species to protect the sowing investment.
- Prioritise paddocks that are likely to be most responsive to lime (paddocks which contain acid sensitive plants).
- Apply lime where nutrient constraints have been addressed or are in surplus.
Because of the need for incorporation, lime application is generally not recommended for native pastures. Some native pastures are very acid tolerant. However, when sowing paddocks with high aluminium levels to phalaris or lucerne, to ensure good establishment, liming should be given higher priority than topdressing other established pastures. From an economic perspective, building soil nutrient levels which are limiting production should come before liming.
Plant response to liming works best when the product is fine (< 0.5 micron), the lime is incorporated into the soil (so moves slowly down the profile) and where the soil surface is acidic, but the subsoil is not. Soils are best limed 2 years before sowing an acid sensitive pasture or crop to enable the lime to fully react or 12 months prior to sowing acid tolerant pasture.
Liming to raise pH also increases the activity of soil organisms, which in turn benefits soil health.
For more information on soil acidity see:
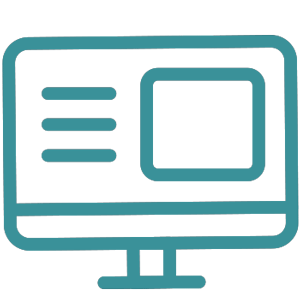
A wide range of fact sheets covering soil health issues from NSW DPI.
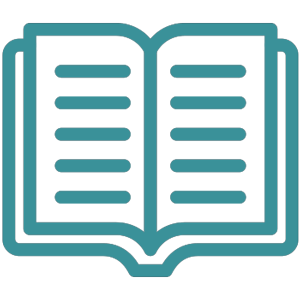
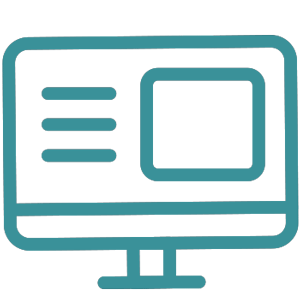
Within South Australia more than two million hectares of agricultural land are susceptible to soil acidification that degrades the soil and reduces crop and pasture growth.
Soil salinity
Soil salinity is usually assessed by measuring the electrical conductivity (EC) of the soil because conductivity is closely related to the level of salt in the soil solution. Most commonly, the EC is measured in a 1:5 soil/water solution (ECw) as this is a much cheaper test, then a conversion factor is applied to account for soil texture.
Table 6.5 shows salinity ratings for a range of soil textures measured by ECw, with the conversion factor for soil texture to convert ECw to ECe to gain a truer picture of plant salt tolerance.
Table 6.5 Salinity ratings of soils measured by ECw, with conversion factor to ECe.
|
ECw (dS/m) |
|||
Salinity rating |
Sand |
Sandy loam |
Loam |
Clay |
Low |
0–0.15 |
0–0.18 |
0–0.2 |
0–0.3 |
Moderately saline |
0.15–0.46 |
0.18–0.55 |
0.2–0.60 |
0.3–0.86 |
Highly saline |
0.46–1.15 |
0.55–1.36 |
0.6–1.5 |
0.86–2.14 |
Extremely saline |
>1.15 |
>1.36 |
>1.5 |
>2.14 |
Conversion factor to ECe |
13 |
11 |
10 |
Light clay 8 Heavy clay 6 |
Table 6.6 Responsiveness of a range of pasture species to soil salinity.
|
ECw (dS/m) |
|||||||
|
No effect level |
30% reduction in growth |
||||||
Species |
Sand |
Sandy loam |
Loam |
Clay |
Sand |
Sandy loam |
Loam |
Clay |
White clover |
0.12 |
0.14 |
0.15 |
0.19 |
0.31 |
0.36 |
0.40 |
0.50 |
Sub clover |
0.12 |
0.14 |
0.15 |
0.19 |
0.31 |
0.36 |
0.40 |
0.50 |
Lucerne |
0.15 |
0.18 |
0.20 |
0.25 |
0.47 |
0.56 |
0.61 |
0.76 |
Cocksfoot |
0.12 |
0.14 |
0.15 |
0.19 |
0.49 |
0.58 |
0.63 |
0.79 |
Tall fescue |
0.30 |
0.35 |
0.39 |
0.49 |
0.74 |
0.87 |
0.96 |
1.20 |
Phalaris |
0.35 |
0.42 |
0.46 |
0.58 |
0.66 |
0.78 |
0.85 |
1.07 |
Perennial ryegrass |
0.43 |
0.51 |
0.56 |
0.70 |
0.73 |
0.87 |
0.95 |
1.19 |
Annual ryegrass |
0.43 |
0.51 |
0.56 |
0.70 |
0.73 |
0.87 |
0.95 |
1.19 |
Conversion factor to ECe |
13 |
11 |
10 |
Light clay 8 Heavy clay 6 |
13 |
11 |
10 |
Light clay 8 Heavy clay 6 |
Managing saline soils
Plants growing in saline soils may face the combined challenge of high salt levels, waterlogging that exacerbates the salinity impact, and extreme grazing pressure (because sheep have a strong preference for grazing salty areas). This makes them vulnerable to overgrazing and erosion and therefore require land class fencing and careful management to keep pasture cover.
Some saline sites will be naturally occurring (classed primary saline wetlands) and support salt tolerant native plants which should be encouraged. Secondary saline sites (formed by rising salty groundwater tables exacerbated by regional tree clearing) may contain salt tolerant species such as spiny rush or sea barley grass and can be sown to improved species.
There is a limited range of pasture species available for soils classed as severely saline, including the legume messina (Melilotus siculus) and Puccinellia ciliata and Distichlis spicata grasses. Tall wheat grass (Thinopyrum ponticum) is suitable for medium salinity. For milder saline sites, tall fescue (summer active cultivars), balansa, persian and strawberry clover are suitable. Saltbushes and burr medic, although salt tolerant, do not tolerate waterlogging that accompanies saline sites.
Out-of-season pasture production can be an advantage on mildly affected saline sites as they usually stay wetter for longer.
The science and technology for establishing and managing saltland pastures has advanced rapidly in recent years but, because saline sites are very variable, it is a good idea to seek local advice from experienced sheep producers or professionals before implementing a program to establish saltland pastures. Tool 5.9 in MMFS Module 5 Protect Your Farm’s Natural Assets contains the current best practice guidelines for productive management of saline land, including some lower cost options for less affected areas.
Areas that are only moderately salt-affected are more financially viable to rehabilitate than areas that are severely affected. Highly saline soils are relatively unproductive even after rehabilitation.
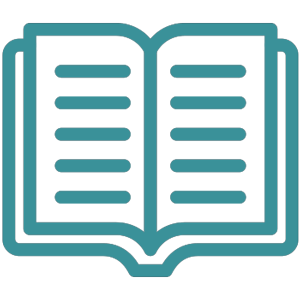
Chapter 10 in the second edition of Greener pastures for south-west Victoria.
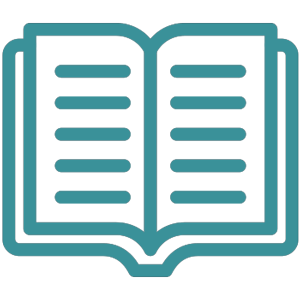
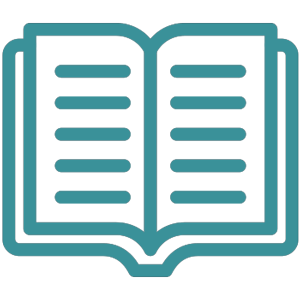
A resource from the Department of Primary Industries and Regional Development.
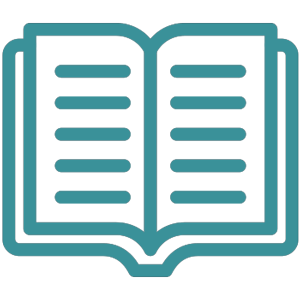
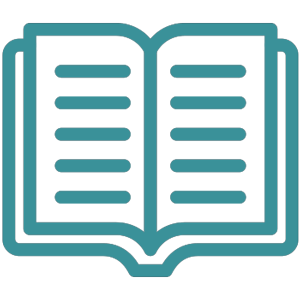
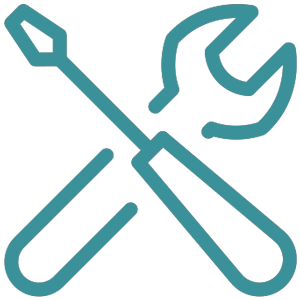
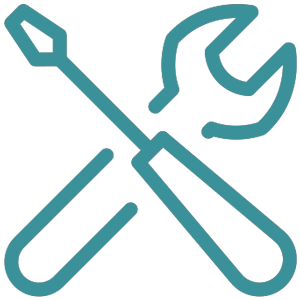
Produced to aid plant identification and help Australian land managers choose likely plants for their situation.
Soil sodicity
In technical terms, sodicity is a measure of the exchangeable sodium percentage (ESP) which indicates how much (percentage) of the cation exchange capacity is contributed by sodium. Sodic soils are unstable because the clays contain an excess of sodium which has weak bonds and so soil particles disperse (clay particles separate from each other). When clay particles disaggregate they clog soil pores that normally contain air and water. This creates soil management problems through low water permeability, surface crusting, waterlogging and clodding. If the soil has good organic carbon levels, this can help bind the soil together but if it is low (less than 2%) then dispersion is likely.
Soils with an ESP above 6% are classified as sodic. Gypsum is commonly added to sodic soils as a cheap source of “soluble” calcium. Unlike sodium, calcium tends to pull clay particles together, so by replacing sodium with calcium on exchange sites, gypsum can stabilise a sodic soil and improve soil structure (Figure 6.20).
Figure 6.20 Calcium in gypsum replaces sodium on clay surfaces and improves soil structure.
If your soil test indicates a high ESP (>6%), you can further test if your soil is likely to disperse and respond to gypsum by placing a soil aggregate (about 5 mm in diameter) in 75 mm of distilled or rainwater and leaving it undisturbed for 24 hours. If the aggregate disperses and the water is cloudy then a gypsum response is possible.
Gypsum rate is determined from the amount of calcium required to reduce ESP to 6. Gypsum rate alters with ESP and the cation exchange capacity (CEC) of the soil because ESP is a function of both exchangeable sodium and CEC (table 6.7). Other factors, such as exchangeable magnesium, organic matter and salt concentration, may modify the response to gypsum, but ESP is the prime influence on soil stability.
Soils with low CEC (<3 meq/100g) and low exchangeable sodium (<0.3 meq/100g) rarely have soil structure problems because they have insufficient clay.
Table 6.7 Estimated gypsum requirement to reduce ESP to target of 6%, assuming gypsum is incorporated to a depth of 10 cm, complete dissolution of gypsum at 70% purity and soil bulk density of 1.5 g/cm3.
CEC (meq/100g) | Starting ESP (%) | |||
8 |
10 |
15 |
20 |
|
5 |
0.3 |
0.6 |
1.3 |
2.1 |
10 |
0.6 |
1.2 |
2.7 |
4.1 |
20 |
1.2 |
2.4 |
5.3 |
8.3 |
40 |
2.4 |
4.7 |
10.6 |
16.5 |
60 |
3.5 |
7.1 |
15.9 |
24.8 |
Generally, rates of 2.5 to 5 tonnes/ha are applied to soil, and better results are seen if the gypsum is incorporated. Gypsum needs to be reapplied at least every five years.
Many subsoils are sodic, but because of high clay content, the sodium displaced from gypsum isn’t leached from the soil and can reattach to the clay particle, so responses are only short lived. In broadscale pasture situations, gypsum is only likely to be economical if surface sealing is occurring and not for treating subsoil sodicity.
Maintaining and increasing groundcover and organic matter levels are the keys to cost effective, long-term management of sodic soils.
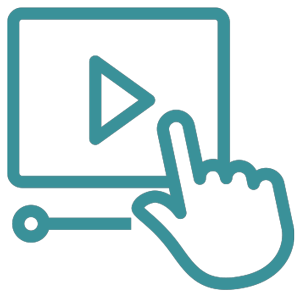
This demonstration shows the effect of calcium (from gypsum) on bringing dispersed soil particles together as aggregates to aid in reclaiming soils with high levels of sodium.
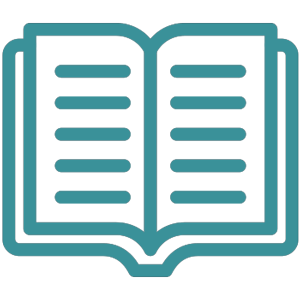
From Tasmania Department of Primary Industries, Parks, Water and Environment.
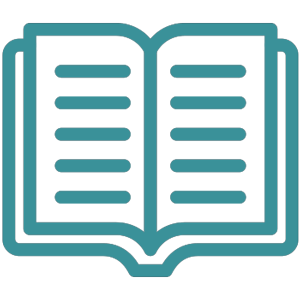
From Corangamite CMA.
Waterlogged soils
Waterlogging occurs when water fills the soil pores and does not drain away, thereby reducing oxygen availability and reducing plant growth. Waterlogging is generally caused by poor drainage as a result of either too much surface water or sub-surface water. It is important to distinguish the source of the water, as surface drains will have little effect if the issue is caused by too much sub-surface water.
Causes of excess sub-surface water are:
- high clay content of subsoils
- high magnesium within subsoils (Ca:Mg ratio is less than 2:1)
- a ‘hard pan’ from excessive cultivation or underlying rock shelf
- rising groundwater.
Waterlogging is most prevalent on lower slope areas, and duplex and heavy clay soils.
Impermeable soil layers can be identified by digging a pit and looking for the following features:
- A bleached layer (pale colour soil at the intersection of the topsoil and subsoil): this indicates that the soil is likely to be waterlogged in winter. It is likely that this part of the soil will be poor in nutrients and most likely quite acid.
- “Buckshot”: small nodules of cemented manganese and iron that have formed under wetting and drying cycles in anaerobic conditions of waterlogging.
- Soil colour of the subsoil clay layer. The following guidelines apply:
-
- A good rule of thumb is that uniform colour down the profile indicates uniform drainage characteristics.
- Red soils are well drained, but if they are waterlogged for a period of time, the iron oxide (which is one of the components that gives soils their red colour) is converted to iron hydroxide which is yellow.
- Mottling (spots of colour) indicates fluctuating/changing drainage characteristics.
- The progression (increasing waterlogging) is from red with yellow mottling to yellow with red mottling to yellow with grey/white mottling to grey/white with yellow mottling.
Plant production losses in waterlogged soils may also result from nitrate deficiency (lack of oxygen leads to soil nitrates being converted into a form that plants cannot use) and fungal diseases (caused by plant roots in waterlogged soils being more susceptible to fungal attack).
Waterlogging effects can be reduced by improving surface and sub-surface drainage, but you will need to carefully consider the feasibility and economics. Elevation mapping and carefully placed drains can catch and divert excess surface water run-off from paddocks into dams. Avoid grazing wet areas as pugging destroys soil structure resulting in less infiltration and pasture damage. Sowing waterlogging-tolerant species such as phalaris, tall fescue, perennial ryegrass, balansa and strawberry clovers can be a solution in some situations.
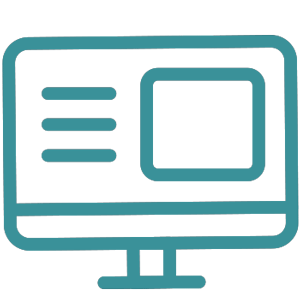
Resources from Agriculture Victoria.
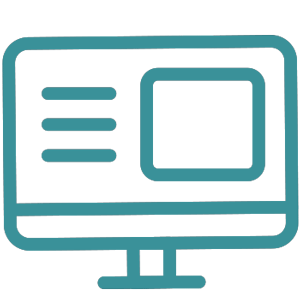
Online resources from Agriculture Victoria.
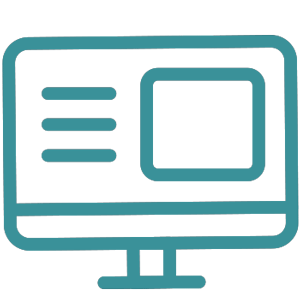
DPIRD WA has a collection of publications and information on the costs, benefits and design of drainage systems for waterlogged areas.
Compacted soils
Soil compaction is the compression of a soil into a hard layer (an increase in bulk density) and is most likely to occur from driving heavy farm machinery, cultivating or grazing cattle on wet soils. Hard pans can also occur as a result of repeated disc ploughing.
To determine if a compaction layer exists, use a spade to examine the soil to about 30cm depth (as you dig you will be able to feel if there is a hard compacted layer) or use a backhoe to dig a deeper soil pit. Compacted soil shows up as a hard, solid layer which can look like bricks rather than loose aggregates. You may see plant roots growing horizontally along the top of the compacted layer or J shaped roots because they cannot grow through it. You can also measure surface soil strength using a penetrometer, which measures the pressure needed to push the rod through the soil.
One off deep ripping of the compacted layer is a solution, but it is expensive and time consuming and needs to be carefully managed if the soil is dispersive. Deep ripping should always be followed up with strategies to avoid any future compaction.
Well managed pastures, especially those with a deep-rooted perennial grass or tap root species (e.g., chicory, lupins or lucerne), will help to break up the compacted soils over time.
For more information on compacted soils, visit:
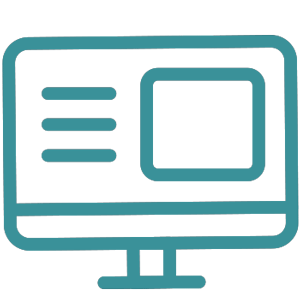
NSW DPIRD
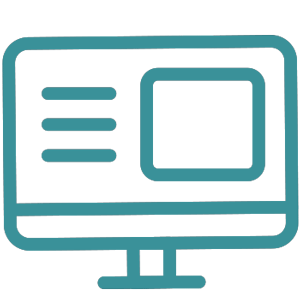
Corangamite CMA Knowledge Base
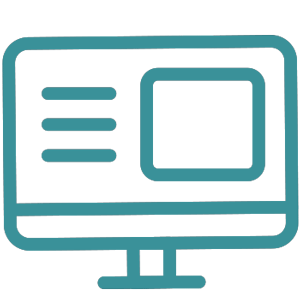
DPIRD WA
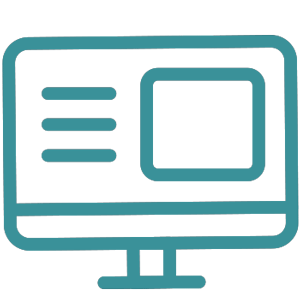
QLD government
Non-wetting sands
Water repellence in non-wetting sands is caused by plant waxes that coat the sand particles and prevent water from infiltrating the soil, particularly when it is dry. The result is poor germination and plant growth and, with large bare areas, it can greatly increase susceptibility to wind erosion.
Figure 6.21 Water droplet on surface of non-wetting soil.
Source: Soil Quality
Techniques to combat the problem include direct drilling, sowing on the contour and sowing into the bottom of furrows with press wheels to improve soil–seed contact and establishment.
Adding clay or cultivating to bring subsoil clay to the surface (delving) has been used successfully, but cost effectiveness depends on clay type and its incorporation into the soil, and on the distance that clay has to be carted from a pit to the paddock. If you think you have non-wetting sand, you can approach a lab and ask for the molarity of ethanol droplet (MED) test to assess severity.
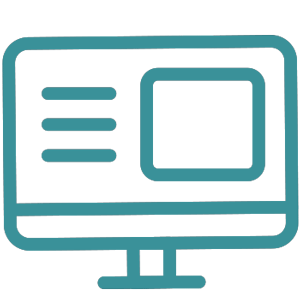
Water-repellent soils have waxes from organic matter on soil particles that cause them to resist the entry of water.
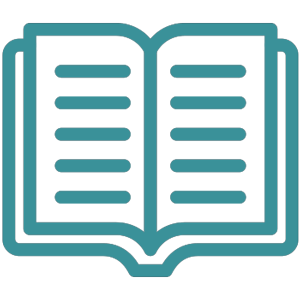
Fact sheet from Soil Quality.
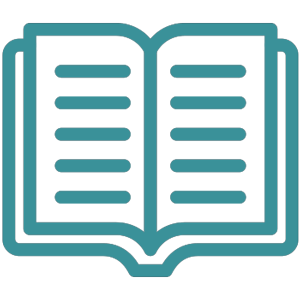
A tour of on-farm research in Western Australia from GRDC
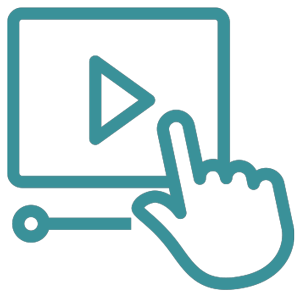
Inspired by what he saw a neighbour doing and with industry research, Corrigin-based grain grower Simon Wallwork bought a mouldboard plough and began a soil amelioration program that is resulting in improved water penetration and better grain yields.
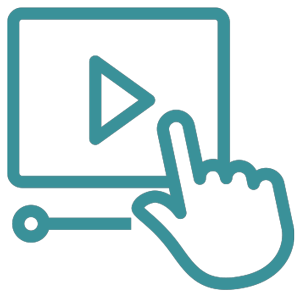
David Hall explains the reasons for non wetting in Western Australia’s sandy soils and its impact on crop production.